Controlling quantumness: Simulations reveal details about how particles interact

In everyday life, matter behaves in a predictable, expected way. If you throw a ball, you assume it will travel in a certain direction and have a predictable recoil. What’s more, forces exerted on one object would not have an impact on another, independent object.
But in quantum mechanics – the physics of the tiny – the rules are completely different. In one, two, and three-particle systems, actions that happen in one spot can strongly influence atoms far away. Scientists don’t yet have a full understanding of this but, by analyzing the behavior of these systems and more complex ones, they are hoping to find insights.
Researchers from the Quantum Systems Unit at the Okinawa Institute of Science and Technology Graduate University (OIST), alongside collaborators at University College Dublin and Durham University, simulated one of these systems, which revealed quantum states – ways that particles arrange themselves in isolated systems – that were unexpected. Their results, published in New Journal of Physics, could have applications for quantum technologies.
“If you throw a stone off a boat, the stone goes one way and the boat goes the other,” explained Professor Thomas Busch, who leads the Unit. “In quantum mechanics, we can have much stronger correlations at much greater distances. It’s like if you put on one red sock and one green sock, then someone in Antarctica, who you’ve never met, would have to do the same. And our work has found new states with these very strong correlations, which can be controlled very well.”
Experimenting with two atoms
When scientists research macroscopic systems, they tend to look at many particles – say 1023. Because there are so many, they can’t follow every atom and must make assumptions. To avoid this, the researchers in this study used another option.
“We simulated a system with just two atoms,” said first author Ayaka Usui, a Ph.D. student in the Unit. “This provided a building block of the larger system, but we could control everything and see exactly what was happening. And, to further control this system, we considered super-cold atoms.”
At room temperature, particles move around very quickly. The warmer it is, the faster they move. By using laser cooling, these atoms can be slowed and cooled down until they reach almost zero velocity and are thus super-cold. This made it much easier for Ayaka and colleagues to describe them in their simulations.
In a system like this, the simplest thing the particles can do is collide with each other. This forces them to move around and change direction, but particles also have something called spin. The spin of a particle is either pointing up or down and further influences how it moves – an effect called spin-orbit coupling. When the researchers simulated a system with two super-cold atoms that were spin-orbit coupled, these new states, with their very strong correlations, were revealed.
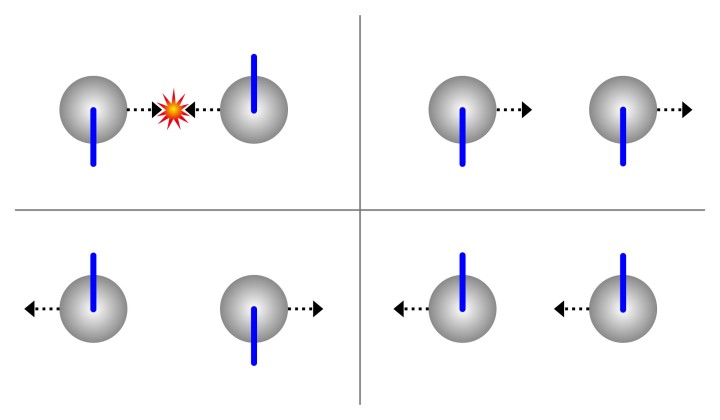
“We have the systems with two-particles where you get these states and the ones with 1023 where you don’t,” said Dr. Thomás Fogarty, Postdoctoral Scholar in the Unit. “Somewhere along this long chain of adding particles, these new states go away.”

Engineering further insights
“Alongside the new states, we’ve discovered the formulas that describe this system exactly,” said Ayaka. “So now, we can engineer it.”
By finding these formulas, the researchers have control over the system and they now plan on changing the parameters to look at the system’s dynamics.
“We’re going to split the system, so we have two of them,” said Ayaka. “We can use the strong correlations to help us measure the system. If we find one atom in one of the systems, we know the other one is also in that one, without measuring it, because they are tightly correlated.”
Although this research is just concentrating on a small aspect of what quantum mechanics can do, it has numerous applications, said Professor Busch.
“Quantum technologies need these correlations,” he explained. “These new states have the strongest non-classical correlations that we know, and we can engineer them. With this research, we could build more powerful computers. We could create measurement devices that measure tiny differences in gravity or electric pulses in the brain. There’re so many applications to work towards.”
Alongside Ayaka Usui, Dr. Fogarty, and Professor Busch, this research involved Dr. Steve Campbell from University College Dublin and Professor Simon Gardiner from Durham University.
Specialty
Research Unit
For press enquiries:
Press Inquiry Form