Space Detectives! Sandbox Craters Reveal Secrets of Planetary Splash Marks and Lost Meteorites
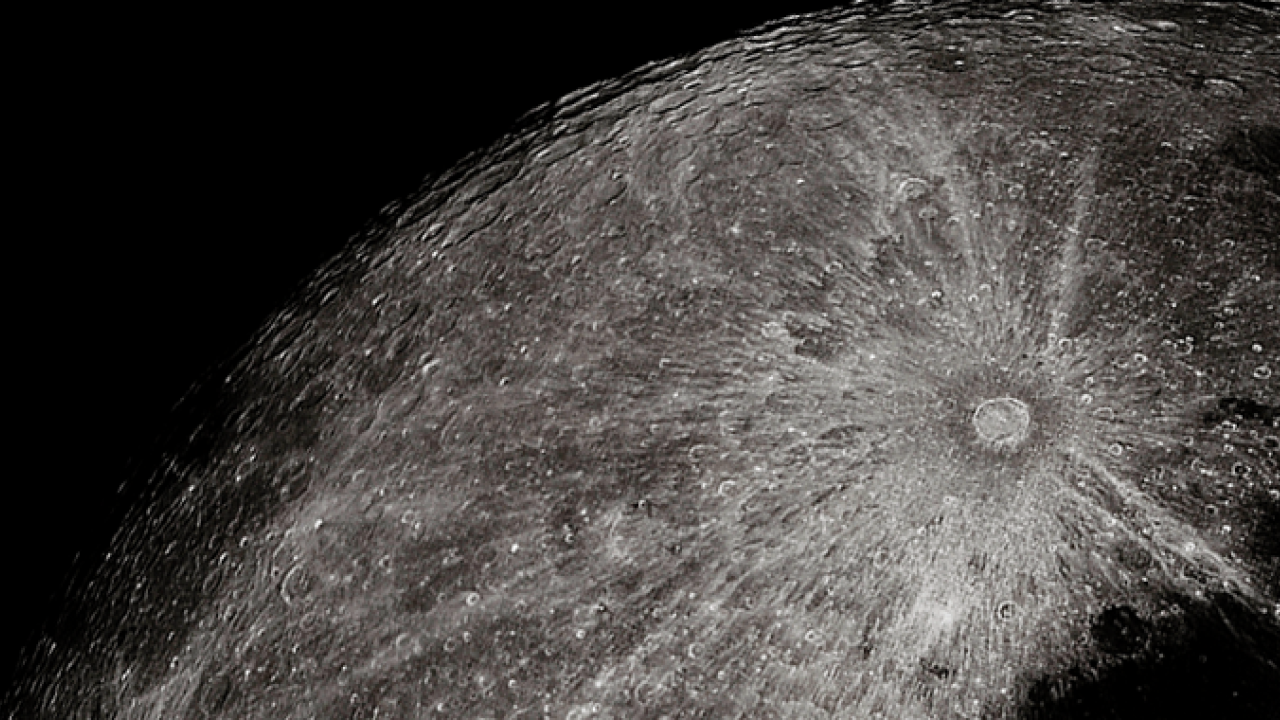
Look up above you. You might see blue sky, clouds, the Moon or stars. And while it might seem calm up there, the truth is it’s nearly always raining. Every day, Earth is constantly bombarded by about 100 tons of falling objects from space, mostly simple dust or sand-sized particles that are destroyed as they hit the upper atmosphere.
But very rarely, a piece large enough to survive the intense heat of entry manages to fall all the way down to the Earth’s surface, where its galactic journey ends with a bump.
Most extraterrestrial rocks that hit the Earth’s surface – meteorites – are so small that they don’t make a dent in the ground. Larger rocks, however, leave their mark in the form of bowl-shaped impact craters. One famous example is the 50,000-year-old Barringer Crater in Arizona, which is 1.2 kilometers across and 170 meters deep. But impact craters have been observed not just on Earth; scientists have also spied them on Mercury, Venus, and Mars, on our own Moon, and on the moons of Jupiter and Saturn.

One feature of craters has puzzled scientists for decades. A meteorite’s force of impact turns the ground into powder and throws that powder high into the air in a cone-shaped trajectory. The flying powder settles around the crater to form a blanket. The question was: why are some blankets shaped like rays -- the long, radial streaks that fan out from the crater’s center like spokes on a wheel?
In a new study published in Physical Review Letters (chosen as an Editors’ Suggestion with an accompanying Viewpoint article), scientists from the Okinawa Institute of Science and Technology Graduate University (OIST) have simulated these extraterrestrial impacts to shed light on how these mysterious crater rays form.
“You can’t make a real crater with a real meteorite,” said Associate Professor Pinaki Chakraborty, leader of OIST’s Fluid Mechanics Unit, “but you can use an analog to simulate what goes on.” An extensively studied simple experiment provides that analog: dropping a heavy metal ball onto a sand bed; the ball hurls out sand forming a crater surrounded by a blanket. “The problem is that these experiments don’t produce crater rays,” said Prof Chakraborty.
But there are some curious exceptions.
It wasn’t until Dr. Tapan Sabuwala of the Continuum Physics Unit led by Prof. Gustavo Gioia was watching ball-drop experiments by high-school students on YouTube one day that he found the first clue about what might be causing the rays: “These experiments are popular in science classes. I noticed that some of their experiments were producing crater rays!”
So what was the unique feature of these experiments? In a word: messiness. Researchers generally even out the surface of the sand bed before dropping the ball, but the videos showed school students performing the experiment, who were skipping that step. Sure enough, when Dr. Sabuwala repeated the ball-drop experiment with an uneven surface, the mini-meteorites made crater rays. “That was the eureka moment”.
It still wasn’t clear why uneven landscapes caused crater rays to form. So the team conducted a second experiment in a flat sand bed imprinted with a regular pattern of hexagonal-shaped valleys. Upon impact, every one of the valleys touching the edge of the ball produced a ray. Christian Butcher, a technician in OIST’s Fluid Mechanics Unit, repeated the experiment with different variables: “We changed the size of the ball, the distance between the valleys, the drop height of the ball, the grains in the bed, and so on,” said Mr. Butcher. The only variables that affected the number of rays produced were the size of the ball and the distance between valleys.
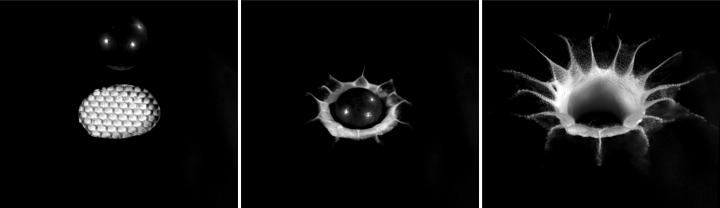
For a closer look at the mechanism behind crater rays, the team turned to computer simulations. “The impacting ball creates shockwaves in the bed,” says Prof Chakraborty. “The shockwaves focus the ejected sand grains from the valleys along radial streaks to form rays.”
Having learned how the crater rays form, the scientists created a theoretical model to predict the number of rays. The model predictions matched well with the mini-meteorite experiments, allowing the scientists to predict what ray patterns would look like on the rough surfaces on real planets.

And there was another exciting twist to their model: it could also be used to learn about the meteorites that have vanished since making a crater. Based on how many rays a crater has, researchers can work out the diameter of the meteorite that created it.
“We can look at nearly any rayed crater with this model and learn about how it was made”, said Prof Chakraborty.
From a simple ball dropped onto sand, the team has created an extraterrestrial lab like in the US TV show “CSI” able to investigate the violent history of the solar system.
--------------------------------
Title Image: Gregory H. Revera
Specialties
Research Units
For press enquiries:
Press Inquiry Form